How do superbugs become super? Understanding antibiotic resistance
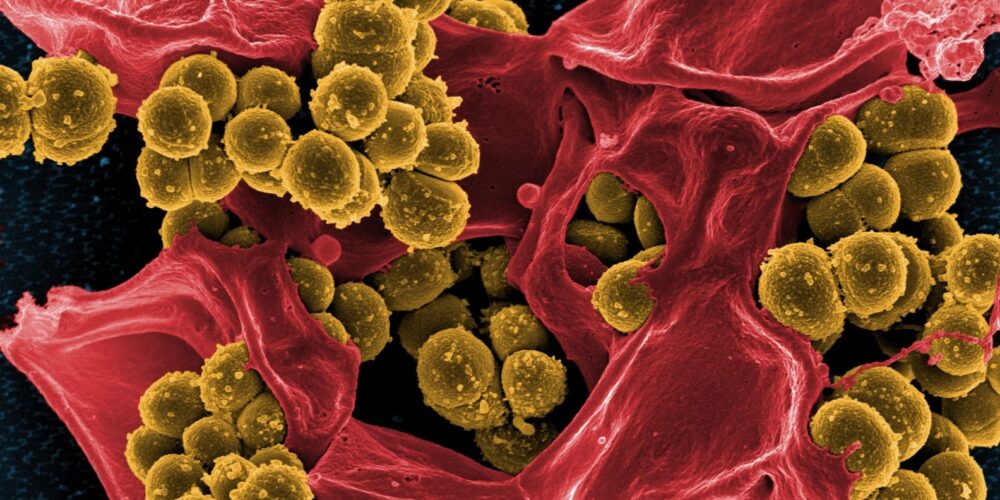
They’re small, but they can be deadly. And we’re still trying to understand how they work so we can protect ourselves.
Antibiotic-resistant bacteria are recognised as one of the greatest threats to human health, and we are rapidly running out of ‘antibiotics of last resort’. The World Health Organisation predicts that if the trajectory of antibiotic resistance continues, bacteria will be killing more people than diabetes and cancer within 20 years. The collateral damage is also enormous. Ineffective antibiotic treatments for infection management may eventually make many medical procedures, from commonplace surgeries to cancer therapies, too risky to undertake.
Dr Josh Ramsay, an ARC Future Fellow in Curtin’s School of Pharmacy and Biomedical Sciences and Curtin Health Innovation Research Institute, is studying how genes transfer between bacteria to help understand how antibiotic resistance evolves.
“Compared to our understanding of 20 years ago, we’ve now got DNA sequencing and other molecular technologies, so we’ve finally got the tools to pinpoint specific genes and trace their movement. It’s letting us identify the mechanisms bacteria use to evolve multiple antibiotic resistance so rapidly”, Ramsay explains.
“we’ve finally got the tools to pinpoint specific genes and trace their movement. It’s letting us identify the mechanisms bacteria use to evolve multiple antibiotic resistance so rapidly”
Bacteria divide to create nearly identical new generations through vertical gene transmission, however, they are now being shown to exchange a surprising number of genes with other types of bacteria through horizontal gene transmission. Ramsay’s NHMRC-funded research with the University of Sydney and University of Western Australia is showing that this mechanism is pivotal to the rapid development of antibiotic resistance.
“Bacteria can develop resistance to an antibiotic through a long period of exposure and evolution, effectively becoming a specialised ‘superbug’ over time. But then in one step, they can transfer all of those genes horizontally to other bacteria. Resistance to multiple antibiotics develops quickly because resistance genes are being collected and shared on transferrable DNA molecules called mobile genetic elements. Since we’ve been using antibiotics more widely, these mobile genetic elements have gradually collected more and more antibiotic resistance genes.”
Ramsay is studying methicillin-resistant Staphylococcus aureus (MRSA), the ‘golden staph’ that has flourished in areas of high antibiotic use like hospitals and nursing homes, but which is now also increasingly being found in isolated community groups. His investigation has been supported by the Antimicrobial Resistance and Infectious Diseases (AMRID) Research Laboratory at Murdoch University, which collects, tests and DNA-sequences bacterial strains causing blood infections in WA. Coming full circle, the AMRID Laboratory itself continues to build on an MRSA database begun by Emeritus Professor Warren Grubb at Curtin in the 1980s.
“That disease surveillance data over time has been invaluable” says Ramsay. “By going back through the old strains, and seeing where these mobile genetic elements have transferred and new resistance has evolved, we have been able to figure out what pieces of DNA transfer, and under what conditions. It’s DNA detective work really.”
A major outcome of his work is an understanding that some genetic elements are independently mobile, whereas others can only move by hitching a ride with a compatible mobile element, if it is in the same bacterial cell.
“It’s a bit like public transport”, Ramsay elaborates.
“The independently mobile elements are like trains, and they can pick up other genetic elements that have the right genes on them, the right ‘ticket’ so to speak, and move them around. One train can pick up resistance genes to a number of different antibiotics, much like passengers on a train. But the train can also help move separate mobile elements together with their collections of resistance genes, much like adding on train carriages. Then all together these large collections of resistance genes can suddenly move together and spread around very quickly.”
MRSA has likely utilised this mechanism to develop even broader spectrum resistance over the years. Treating it compounds the problem – it doesn’t matter if you use an antibiotic of last resort or the classical penicillin, evolutionary pressures select for the bacteria that contain ‘trains’ and their load of resistant genes, simply because they are the most rapid evolutionary path to resistance. If those mobile elements happen to confer resistance to Linezolid or penicillin, they flourish regardless, and rapidly confer any other resistance genes they are carrying into the bargain. Prolonged antibiotic usage is indirectly selecting for more advanced gene-transfer infrastructure.
The use of antibiotics is a true double-edged sword. If you need to take antibiotics to manage an infection, all of the bacteria in your body are exposed to it, not just the ones causing illness. And any developing resistance can be translated into those populations. Even the antibiotics used in agriculture to manage animal health eventually select for these ‘trains’, allowing any antibiotic resistance that has developed through years of animal treatment to spread between bacterial populations, including to those that prefer human hosts. It becomes apparent that any rational approach to antibiotics management has to consider not just the human population, but also our companion animals and our entire food chain.
Understanding how resistance genes transfer across bacterial populations, in combination with ongoing antibiotic resistance monitoring, is now starting to inform recommendations for antibiotic prescription and management of infections. The faster doctors can determine which antibiotics to avoid and which might be useful to treat specific bacterial strains, the better the patient outcomes while minimising the development of additional antibiotic resistance.
“We’re really only now starting to understand how these bacteria really evolve”, says Ramsay. “But the better we can understand the mechanisms of gene transfer and how resistance has evolved in the past, the more we can predict how bacteria are likely to continue evolving. This understanding also informs strategies for better use of our current antibiotics, and opens new avenues to combat resistance. If we can develop ways to interfere with these gene transfer mechanisms, we could silo pockets of bacterial antibiotic resistance, stop their spread, and slow the evolution of new superbugs right down.”
The end goal may still be a fair way off, but Ramsay’s fundamental research investigating how superbugs become super, at a molecular level, is a necessary first step in understanding bacteria and breaking the cycle of antibiotic resistance.